Geopolymers are being researched as alternative materials to conventional cement (Portland cement, PC) in the construction industry due to their lower CO₂ emissions during manufacturing. Given their chemical composition, which resembles certain subsurface formations, we propose that geopolymers may be applicable in geothermal reservoirs to improve permeability and thermal conductivity, both critical for effective thermal energy extraction. The aim of this research is to investigate the solidification behavior of geopolymers and identify the dominant factors influencing their chemical structure evolution. This understanding could enhance the potential for utilizing geopolymers in deep geothermal energy applications. Recent studies on Enhanced Geothermal Systems (EGS), which create artificial geothermal reservoirs through advanced drilling and subsurface water circulation techniques (Fig. 1), have gained significant attention. EGS can be categorized into two approaches: closed and open-loop systems. While open-loop systems may extract more thermal energy, they pose challenges in controlling water circulation paths. We hypothesize that geopolymers could be used as foundation improvement materials in EGS, given that they remain in a liquid state until the solidification process progresses, making them suitable for injection into the foundation.

The solidification process of geopolymers differs significantly from that of conventional Portland cement (PC), presenting challenges in understanding its behavior. PC, primarily composed of calcium hydroxide or calcium silicate, undergoes solidification through the formation of crystal phases. In contrast, geopolymers, derived from aluminum silicate, solidify through the evolution of an amorphous phase. This fundamental difference introduces complexity in the study of geopolymers, as amorphous structures are more difficult to analyze using conventional chemical structure techniques. X-ray diffraction analysis (XRD) is commonly used to identify the chemical structures of cement materials. In the case of PC, the XRD spectrum shows sharp, distinct peaks corresponding to the crystalline phases. However, for geopolymers, the XRD spectrum is characterized by broad peaks, indicating the presence of an amorphous phase that develops over the curing period (Fig. 2). These broad peaks complicate the process of determining the precise quantities or positions of peak tops, highlighting the inherent difficulty in identifying the dominant factors that govern the solidification of geopolymers.

However, we infer that the evolution of the chemical structure is more critical to the properties of geopolymers than the chemical composition itself. Figure 3 illustrates the compressive strength behavior of two types of geopolymers with identical initial geometry and similar chemical compositions but differing in crystal/amorphous phase content. The brown-colored geopolymer exhibits high strength but is brittle, whereas the white-colored geopolymer shows lower strength and undergoes plastic deformation. These results suggest that factors other than chemical composition play a dominant role in determining the mechanical properties. Therefore, we aim to establish a correlation between the evolution of the amorphous phase and physical properties such as compressive strength and thermal conductivity.
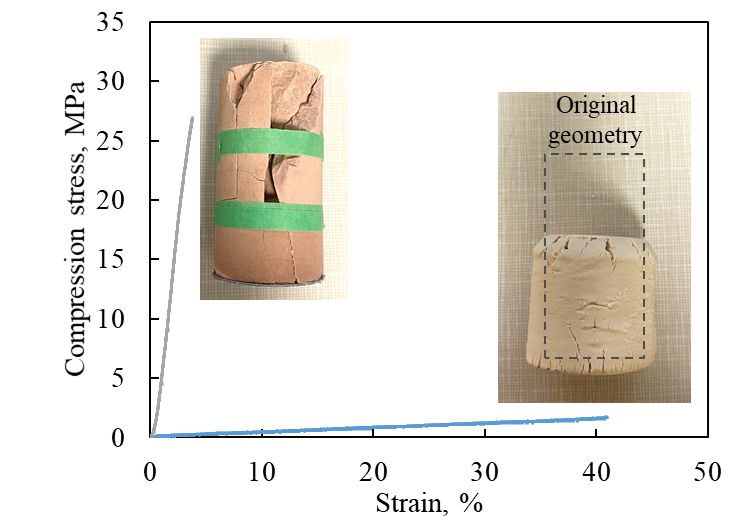